What is “Sandwich Construction?”
One of the biggest benefits (“superpowers!”) of composites is the option to use “cores” to create a structure with variable density across thickness. Thinking about the bending of a piece of material, it is intuitive that thicker pieces of the same material will be stiffer in bending than thinner ones. The problem with thick things is that they are heavy – and only the material out at the edges is bearing much tensile or compression load under bending. Cores let you replace that middle bit with a much lighter material that is there only to transmit shear and compression loads between the surfaces (aka: “skins”, “face sheets”). This core keep the sides apart and keeps them from sliding by each-other. This is called “sandwich construction” or “cored construction” because the thin layers of reinforcement “sandwich” a thicker and much lighter core material. This way you get the stiffness of a thick piece, with the weight of a thin piece!
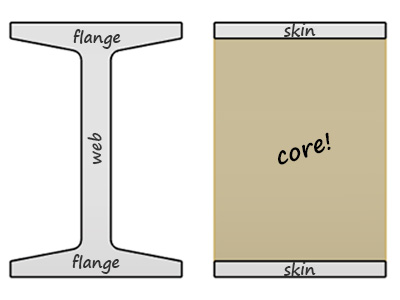
You can think about a cored composite panel in one axis as like an I-beam. The web of the I-beam is there to keep the flanges apart and to keep them from sliding by each-other. With sandwich construction in composites, the core serves as the “web” of the composite structure.
A panel has this going on over a surface, instead of a single axis like a beam. Because there are so many variables, it can be hard to engineer composite panels. Cores have a wide variety of shear and compression properties. Skin thickness, fiber orientation and material type all play important roles in the properties of the panel as a whole. To top it off, bonding the skin to the much lower density core is sometimes a challenge!
This article is about core basics and how to consider cored composite design in a no-math kind of way.
Why Core?
Here are two panels from the materials library (#7 and #10). They are both made with with e-glass and epoxy resin. They weigh about the same amount. One has core, the other doesn’t.

I put a big heavy chunk of aluminum on both of them when they were supported by their edges. The single-skin panel deflected a lot – the cored one didn’t. Sure, it deflected a little – but not that I could see!

Those panels illustrate the huge benefits of sandwich construction for composite panels. If the ability to point reinforcement in any direction we want (anisotropic) is composites superpower #1, then superpower #2 is the ability to push the structural elements out to the edge of thick panels using a light weight core. Core makes so many of the high strength-to-weight benefits of composites possible!
More Stiffness, Not Much More Weight
Imagine we’ve got a single-skin panel – 1/4″ (6mm) of resin and woven e-glass. It’s heavy and strong, but not particularly stiff. Here’s what happens to the stiffness of that panel if we split it into two 1/8″ (3mm) skins and put some core in the middle. The stiffness goes way up as we add thickness. Doubling the thickness of the panel with 1/4″ (t=.25) of core results in a panel that is seven times stiffer! Here’s a graph:
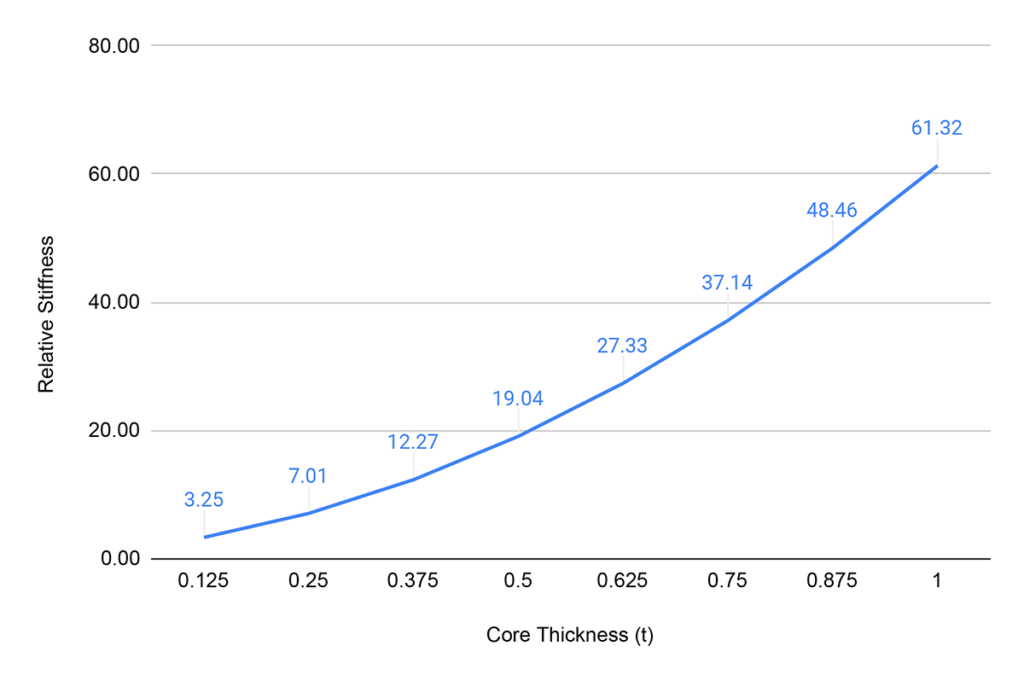
Now stiffness is awesome and all, but the real awesomeness comes when we look at the weight too. As thickness goes up, so does weight for a single-skin panel – in a 1:1 way. For a cored panel, that extra thickness comes only at the expense of the core weight – and cores are usually very light!
A typical density for e-glass/epoxy laminate might be 1600 kg per cubic meter. For a foam core, 80-200kg per cubic meter is fairly standard. The core is a tiny fraction of the density, and yet it can be engineered to provide the compression and shear strength needed to keep very thin reinforcement “skins” where they belong.
Here is a chart for that same example panel comparing the weight of a cored panel to a single-skin one of the same thickness. The base panel at 1/4″ thick weighs 1 unit. At 1/2″ thick, the solid panel weights 2 units, while the cored panel weighs only 1.1 (assuming a core that is 1/10 the density of the solid laminate) units. Comparing this to the stiffness graph above for the same material, it is easy to see how quickly cored panels achieve impressive stiffness-to-weight ratios.
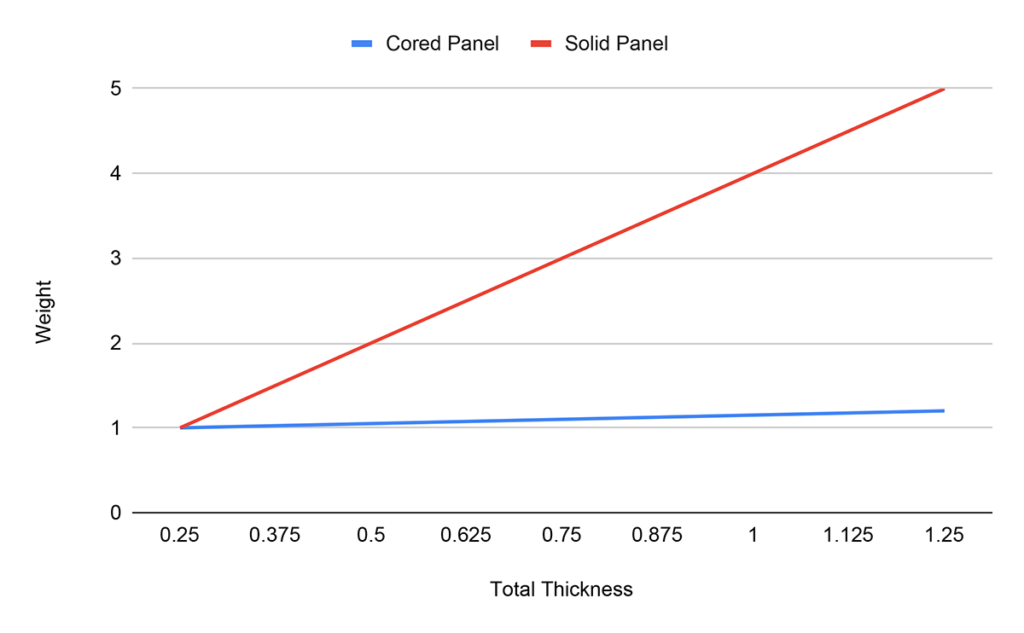
So for those panels in the pictures in the last section – the ones with the big aluminum block bending them – the cored one (1/16″ skins on 3/8″ core) is about 40 times stiffer than the single skin 1/8″ thick panel. Not bad for the same weight!
Why NOT Core?
It’s not all sunshine! Cores bring plenty of additional structural and manufacturing challenges to your part. For some types of structures they are more trouble than they’re worth and a thicker single skin panel or a panel reinforced with structural members like ribs, stringers, and bulkheads may be a better choice.
One of the big downsides of core is that the face-sheets or skins are thin and prone to damage and de-lamination. In the case of impact, the core can become dented and pull away from the skin, or worse, the skin can break and expose the weak core only supported by the remaining skin. Sometimes this kind of failure can be catastrophic and will result in rapid spread of damage under loads or flexing. Once a skin comes un-stuck from a core, the shear loads aren’t transmitted anymore and the laminate loses its stiffness.
Cored structures are also more complicated to build. The detailing for core around joints and holes leads to not-obvious load conditions as stresses in the skins come together in less-than-graceful ways. Extra care is needed to make sure that edges are capped or reinforced with flanges. Core bonding can be problematic with the potential for voids or un-bonded zones. Foams, woods and honeycombs all have areas where things can go wrong and it isn’t always easy to see the problems without extensive destructive or non-destructive testing.
So… that’s only a little overview of the potential problems that cores bring along with the light weight and super stiffness. As always with composites – no free lunch!
Different Core Materials
When it comes to choosing a core, you’ve got a whole bunch of options. Not only are different materials available, but for each material, a wide variety of densities are common. Foams used as cores come in densities as low as 30kg per cubic meter and go right up to 200kg per cubic mater and beyond. Honeycombs are even more complicated, because they have density, cell size AND the orientation of the material to consider. More on honeycomb later. For starters, here are some pictures of the core materials we’re going to discuss:
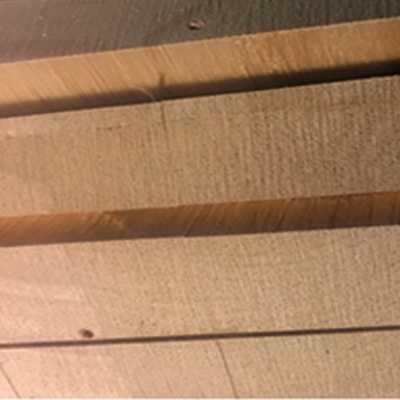
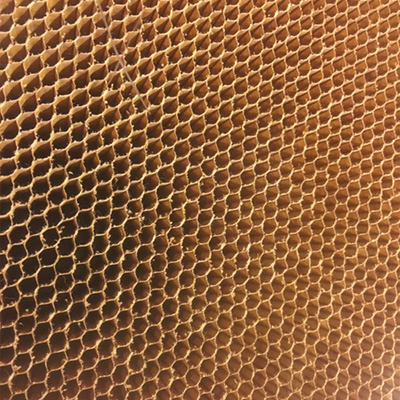
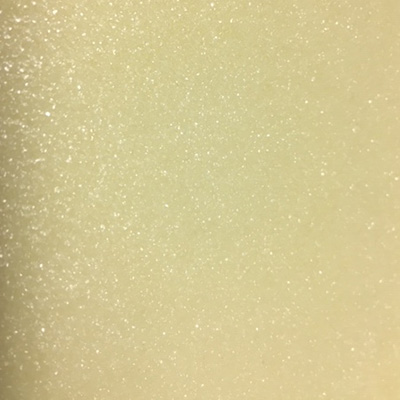
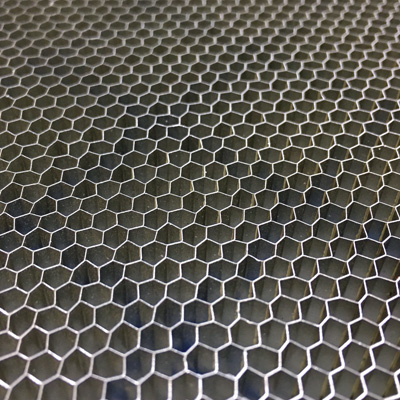
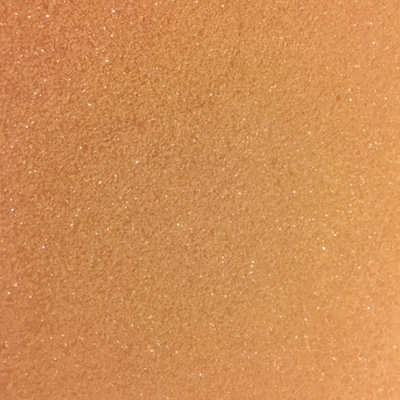
End grain balsa is just the wood of a balsa tree sliced into thin slices and arrayed together side-by-side. The grain of the wood runs in the thickness direction for maximum compression strength. Balsa is graded by density of the wood itself. It can be bought in contour-cut sheets made of small blocks glued to a scrim (for curved surfaces), or in solid sheets where the small blocks are edge-glued together into a sheet.
Nomex honeycomb is made of aramid (Nomex is a DuPont trademark – but it is somewhat generic in use) paper with resin applied in strategically placed strips. Wide sheets of this partially glued Nomex paper are stacked up and pressed together. When the glue has cured, the sheets are pulled back apart (“expanded”) , with the glued surfaces of each adjacent sheet staying stuck – forming an hexagonal honeycomb-cell shape. The edges that are glued together are doubled up – and are all parallel so the shear strength in this direction is much higher. This is called the “L” or “ribbon” direction. The other (90 degrees from “L”) is called the “W” direction. The honeycomb paper is then dipped into a phenolic resin and this resin is allowed to cure before the billet of honeycomb is sliced into sheets. Density is controlled by the thickness of the paper substrate, and the amount of resin the honeycomb is coated with after expanding.
Gurit Corecell M-Foam is a SAN (styrene acrylonitrile) foam, and DIAB Divinycell H is a PVC (polyvinyl chloride) foam. Both are very common materials and offer similar properties. This isn’t science, but my feeling is that though mechanically the Divinycell is slightly better, the Corecell is tougher. Certainly it feels this way when you handle them. In my experience, they are often used interchangeably at similar densities. The Corecell M-foam can be used successfully with low-temperature-cure pre-pregs though it is not very dimensionally stable compared to higher performance (PEI or PMI – like Rohacell) foams.
For more core examples, see Core Material Reference in the Materials Library Section.
Mechanical Properties of Core Materials
Core materials face two main types of loads: compression and shear. Compression loads are squeezing the core together – trying to crush it. These come from outside pressure and impacts, but mainly from the two skins “wanting” to get closer together as bending loads are applied to the panel as a whole. Shear loads are caused by the difference in the tension and compression faces – the pressure trying to slide one skin by the other. Here’s a picture:
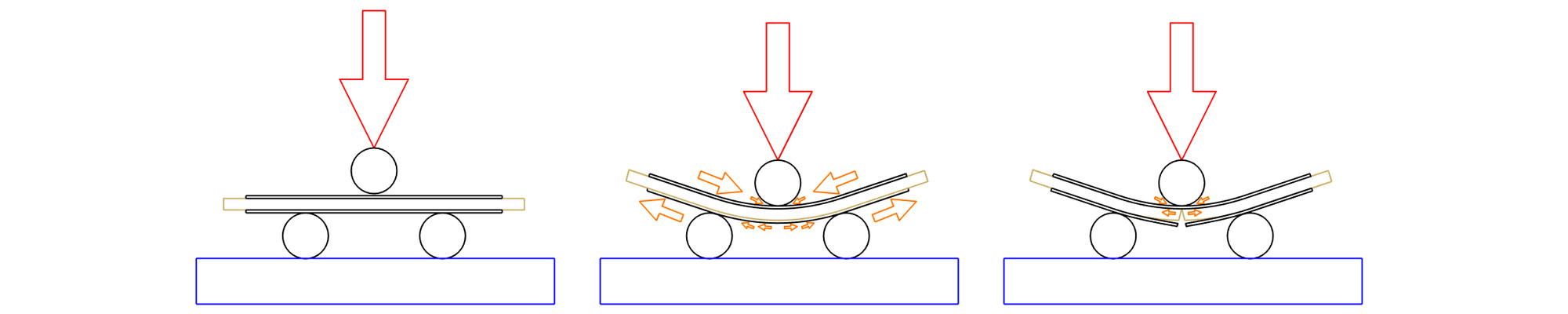
The panel starts off with no bending load on it. As the arrow comes down and bends the sandwich panel, the bottom skin is “asked” to get longer and the top skin is “asked” to get shorter. The core is in compression where the three cylinders bear on the panel. The core is being pulled “in” to the middle on the top skin and pulled “out” on the bottom skin. This difference in direction top to bottom is called “shear” loading. The load is trying to make the core slide along the skins in different directions. The skin-to-core bond needs to be able to handle this shear load, and the core itself has to resist splitting parallel to the skins. Here the load is too much for the bottom skin and it fails in tension. The core splits too because it was bonded to the bottom skin. Now the broken panel is still bending, but the shear is now only in the much more flexible top skin.
This is not meant to be a realistic scenario – only to explain the nature of compression and shear loads faced by core materials. I’m going to work on making better illustrations of this. Now lets look at some materials commonly used for cores and check out their relative mechanical properties:

Now why would you ever use foam, if the compression strength of Nomex and balsa are so much better? Well, chances are you don’t need all that compression strength for most things. If it isn’t necessary, why pay more (or weigh more) for it? Foams also handle impact more gracefully and don’t have as much variability in processing as balsa – and can be used with simpler manufacturing methods than honeycombs.

And here again, with shear you can see that the Nomex in the “L” direction is pretty awesome for it’s density. This is why you find honeycombs (aluminum is even better) in aircraft, racing cars and boats and all kind of other items that are fast, light and… not cheap! Processing honeycombs is almost always done with pre-pregs which add a huge level of expense and complexity to the manufacturing process. It’s worth it for some high-performance projects, but in most situations it’s not worth the money.
Striking a Balance
So you can see how great cores are for making really stiff lightweight composite structures. But how do you design for successful fabrication and take advantage of mechanical properties without running into other problems? Engineering cored composite structures is not a simple process. I am not qualified to explain it with engineering level rigor – though I will try to cover basic topics and trade-offs. Lets just look at core-related considerations without any numbers or formulas attached…
Your goal is to strike a balance between the core and the skins so the laminate as a whole does what it needs to do. Skin strength, stiffness and thickness is played out against core (compression and shear) strength and density as the panel is subjected to loading conditions. Different conditions will place more or less demand on various parts of the structure. Your composite panel has got to meet the demands of both the structure as a whole, and as a panel locally. For example, a boat needs to handle the load from the engines (or sails) driving the whole thing forward, which is a global load issue, and at the same time, the bottom of the boat needs to absorb and transmit the loads from slamming up and down on waves. The deck of the boat needs to handle impacts from people walking around (and partying in high heels?) One situation places loads on the structure as a whole – bending, twisting – big picture stuff. The other places loads locally on areas of the cored panels. Both requirements must be met.
The other type of harmony needs to be met within the panel itself. If you have very light skins on a thick high density core, the skins will break or buckle way before the core even starts to stretch it’s legs – so you’re carrying around unnecessary weight. On the other hand, if you have thick carbon skins on some lightweight honeycomb, the core might crush/shear/go to pieces before the skins absorb even a small fraction of their potential in a bending situation. This balance can be hard to nail down because of the variety of demands on a structure, but in general as the skins get thicker, the core should get more dense. Keep in mind that many structures are built with a variety of core densities and even types in a single panel or part. Sometimes core is eliminated entirely in highly loaded or mechanically fastened areas.
Global structural loads are beyond the scope of this article. In many cases, a cored panel will meet these automatically just by being stiff enough to handle local panel loads. For heavily or “complicatedly” loaded parts and assemblies, serious engineering is necessary. If you have doubts, find a structural engineer experienced with composites to help you out.
Test Panels
One of the most useful things you can do if you are designing a composite structure (especially a cored one) is to make test panels. When you are working from material datasheets and formulas there is a big “can this be made like this and how will it actually work” hurdle still to come. Making test panels is a great way to validate the theory with some reality. Flat panels are easy to make and can be tested as strips to get linear properties (beam style) and also as panels with multi-axis properties. Things like core shear and compression, skin thickness to core thickness ratios, and skin/core bonds can be tested experimentally to make sure that there’s no big “oops” hiding in an assumption or a proposed manufacturing method. Sometimes it is necessary to “break up” cored panels by testing samples of skin laminate alone to validate their properties and then consider the core-related testing separately.
The Laminate Samples section of the EC! Materials Library is full of examples of test panels. Just cutting strips of different samples and bending (and breaking!) them will give you a feel for what you have and how it will work in practice. Hitting samples with a hammer or dropping weights on them can help you see how different skin thicknesses and core materials behave when damaged. It’s also fun!
Keep in mind that even with a non-scientific seeming approach (hammers, breaking samples with your hands, etc.) it is good to apply as much control as you can to eliminate variables. Treat each sample the same – cut strips the same width and in the same orientation, drop the weight from the same height, hold the panel the same way for each test… you’re still doing science even if you don’t generate numerical data!
Depending on your engineering skills and access to testing equipment you can go nuts with this – and in many situations this is totally appropriate. Provided you are comfortable with your assumptions, time and money spent testing is rarely wasted. It is very discouraging to build something big and expensive and then to have to throw it out or make serious modifications because you didn’t get the basic engineering right from the start. I said it before and I’ll say it again – when in doubt get professional help! Or at least copy somebody else who has built a similar and successful item to start with.
Failure Modes – And What They Mean
This part is going to get written soon with pictures and a video about this because it’s really easier to see.
Core Concepts
I hope you take at least these three things away from this overview:
- Cored “sandwich construction” is awesome for making light and stiff panels. It may be the best way there is!
- Core properties need to be balanced with skin laminate properties to make a panel where all is harmonious(ish).
- Make test panels! Break them like a scientist.
And number four – seek qualified and experienced help when designing high-stakes composites structures. If people are going to ride it it, walk under it or get hurt if it fails – it’s super important to get it right!
Additional Resources
Please send me any links to resources that you think should be included here. Thanks!